In 2014 there were 3634 outages in the US electric system according to the Eaton Blackout Tracker. It affected in total over 14 million people. On average close to 4000 people were affected per outage, which on average lasted 43 minutes. 30 % of the outages were caused by weather and trees. 28 % were caused by faulty equipment and/or human error.
Almost all outages were at the distribution system level, Outages at the transmission level are very rare, but when they happen the consequences are bigger, affect more people and take longer time to restore. The Northeast Blackout in August 2003 hit 55 million people in United States and Canada. One month later the Italy Blackout had also about 55 million people in Italy, Switzerland, Austria, Slovenia and Croatia losing power. As recent as in March this year 90 % of Turkey with 70 million people lost their power. The largest blackout so far was in July 2012 affecting half of India and 620 million people. In fact the grid collapsed for a second time in two days.
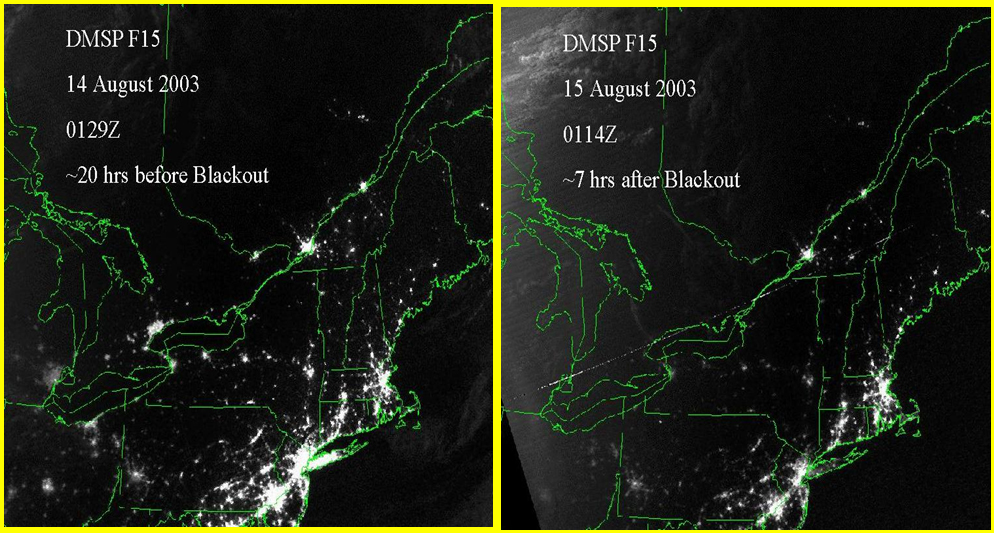
2003 Northeast Blackout. Satellite pictures before and after the blackout. National Geophysical Data Center (NOAA/DMSP).
These large blackouts are the result of cascading events, originating from a single point of failure. For example if a failure happens on a fully loaded or an already overloaded transmission line and the line disconnects, it can result in overloads of neighboring transmission lines, causing them to trip. In turn it leads to more lines to be overloaded and trip. Once the cascade has started it goes very quickly and faster than any operator can react. Eventually the grid breaks up. How it breaks up depends on the actual situation. It has not been possible to design a very large electric grid in the case of a collapse to break up in a structured way into predetermined islands with a balance of supply and demand. After all protection relays are set to protect equipment (power plants, transmission lines and substations) not the system!
Several measures to make the grid more robust have been made and continue to be made. After the 2003 blackout The North American Electric Reliability Corporation (NERC) added to its reliability standards a N-1-1 criteria, which means that the system has to be designed to withstand not only a loss of a major generating unit or power line, but also withstand the loss of an additional generating unit or transmission line. The N-1-1 criteria set a high reliability standard, which is achieved by adding transmission capacity and/or building redundancy.
All these planning and compliance measures help to further reduce the risk for a large blackout. However, new threats to system have been added, such as extreme weather, physical and/or cyber-attacks. The argument has been made that the very large electric grids are becoming too big to fail. From a reliability and resilience perspective instead of one very large system it would be better to have several smaller but still large systems.
Two of the largest systems, if not the largest, in the world are the Eastern Interconnection with a production capacity of over 738 GW (projected for 2017) serving 217 million people in North America and the Continental Synchronous Area with 667 GW (2009) production capacity serving over 400 million people in 24 European countries. These and other very large electric grids offer huge economies of scale. The larger the footprint the more one can optimize a variety of generation resources and a variety of load demands.
Looking forward the value of very large grids will further increase with more variable renewable generation (wind and solar) being brought on to the systems. In fact it has been shown by countries like Denmark and Germany that without strong transmission ties to neighboring systems, it would not have been possible manage the large of wind and solar. (See “The Importance of Strong and Nice Neighbors. Europe.”) In Europe looking at substantially expanding renewable energy sources, not least offshore wind, there are discussions about an European Super Grid expanding the existing system to Africa, Kazakhstan and Turkey. Here in the USA several studies, for example by Department of Energy’s (DOE) National Renewable Energy Laboratory (NREL) have come to similar conclusions.
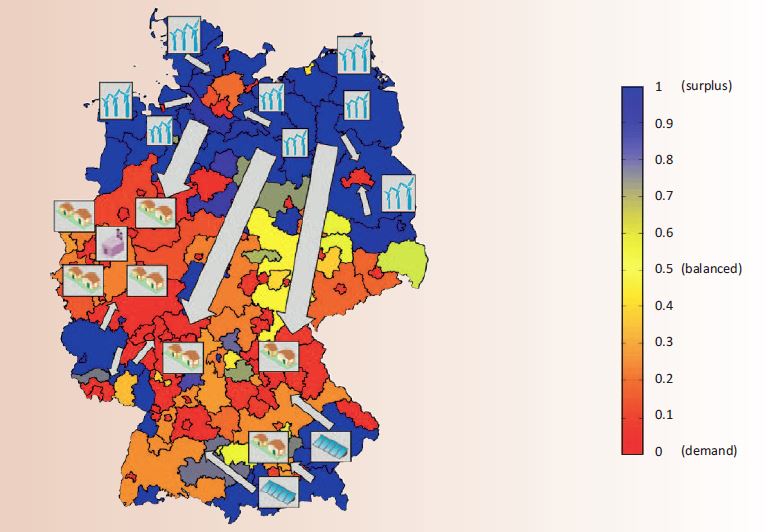
Germany. The rapid growth of wind generation in the north and more solar in the south coupled with closing of the nuclear power plants have caused a major balancing challenge not only in Germany but also for neighboring systems. The need for 3 – 4 HVDC transmission lines have been identified as the preferred long-term solution.
Source: IEA Technology Roadmap. Energy Storage referring to a 2013 report from Fraunhof Institute.
Thus the challenge has become how to develop very large electric grids to capture the economy of scale, while further reducing the risk for blackouts as well as strengthening the resilience. It starts with getting a more precise view of the whole system. Phasor Measurement Units (PMU) brings a step function improvement in that respect. Conventional technology measures voltage, current and frequency in about every 4 seconds. PMUs measure 30 times per second. Further the data is time stamped. Thus PMUs from different locations are synchronized. It enables much more accurate visualization of the status/health of the whole system. Bottom-line it can give operators “early warnings” and more time to take actions to maintain reliability.
Nevertheless, the key to achieve a more reliable and resilient system may be the topology of the system. In a traditional grid with HVAC (High Voltage Alternating Current) the electricity will flow on the path of least resistance. In some cases phase angle regulating transformers (PAR) are used to force the power to flow defined ways, but overall the degree of controllability is very limited. On the contrary HVDC (High Voltage Direct Current), which historically has been recognized for being the most cost efficient way for long-distance bulk power transmission, offers significantly more controllability. The amount of power transmitted by a HVDC line can be ‘throttled’ from full power to idle and the direction of the flow can be reversed very fast.
The newest HVDC technology, Voltage Source Converter (VSC), takes controllability to the next level. VSC can operate independent of the surrounding HVAC system. It can also provide reactive power for voltage support. In addition VSC has black-start capabilities, which is significant in the restoration after a black-out.
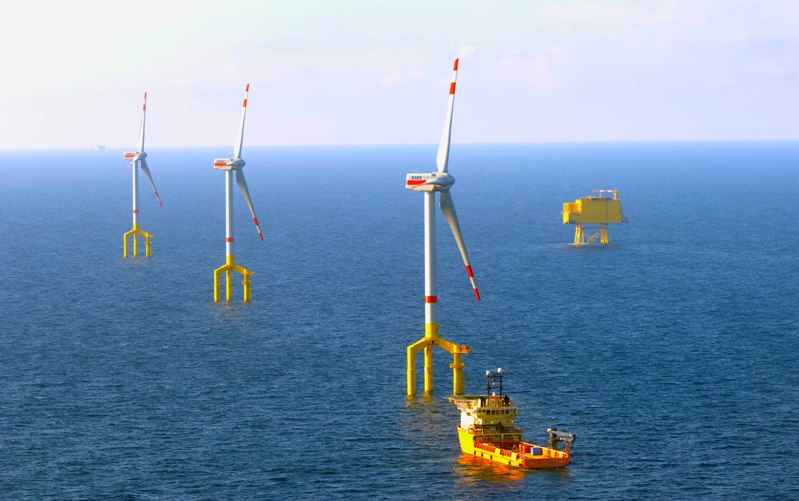
BoWin 1. 80 wind generators each at 5 MW located in the North Sea 130 km from the coast. The 400 MW of power is brought to shore by HVDC VSC. This technology was pioneered by ABB and originally intended as a distribution system level HVDC and thus was called HVDC Light. The first commercial project was for a 30 MW windfarm on Gotland, Sweden. in 1994. The technology has quickly been scalled up to the transmission level and VSC can now handle over 1000 MW.
Europe has become a leader in using HVDC, both classical and VSC. Most of the recent expansions between European countries have been by bilateral connections using HVDC. In a similar way the reliability and resilience of the Eastern Interconnect would benefit from more HVDC, especially with more variable resources connecting to the system. Presently Eastern Interconnect has several hundred (HVAC) interconnections between the various control areas of the system.
One of the most intriguing electric infrastructure projects in North America is Tres Amigas, which will use HVDC back-to-back to connect all the three US electric systems: Eastern Interconnection, Western Interconnection and Texas Interconnection. Fully expanded it is intended to be able to transfer 5000 MW, which would not only be most significant in economically balancing the systems with increasing amounts of variable resources, but also enhance the reliability and resilience of all the three systems. A couple of thousands MW can be the decisive difference in an emergency situation to keep the system up instead of collapsing.
In the rare case of a cascading black-out, most likely the HVDC connections will help in a more structured break-up of the system, even though it still will not be in a perfectly controlled manner. A new concept, microgrids, may be the better answer.
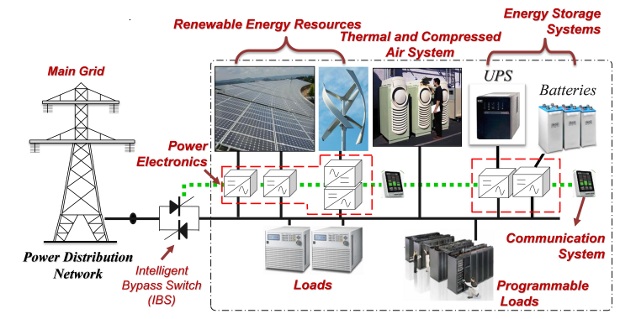
Microgrid schematic.
Source: Picture from an article: “Microgrids. Independent Local Power Grid.” in Tech The Future March 6 2014. The article was first published in Elektor Magazine December 2013.
Microgrids hold a lot of promise to provide a tool for a controlled way to island parts of the system instead of shedding load and that way avoid a major failure on the system. (See “A Perfect Storm For Microgrids.”) Also in the case of a large blackout microgrids could help speed up the restoration. However, to be significant and meaningful from a very large system perspective the aggregated amount of microgrids will most likely have to be in the thousands of MW. With microgrids ranging from 1 to 100 MW it would mean several hundreds of them.
The ultimate vision is a highly reliable very large grid with self-healing capabilities. We may never get there, but PMUs, HVDC and microgrids will help us to move in the direction of such a vision.